Revolutionizing Orbital Mechanics Through Advanced Engineering
The Evolution of Modern Spaceflight Engineering
Modern spaceflight engineering has transformed orbital mechanics through the implementation of controlled chaos principles. By harnessing gravitational resonance and non-linear dynamics, engineers have achieved remarkable 27% fuel efficiency gains compared to conventional orbital trajectories.
Advanced Technology Integration
The integration of quantum-enabled sensors and sophisticated machine learning algorithms has revolutionized orbital calculations, processing data at microsecond intervals to ensure precise navigation through complex gravitational fields. These systems maintain unprecedented levels of accuracy in orbital trajectory management.
Precision and Prediction Systems
Monte Carlo simulations now enable trajectory predictions with 99.7% accuracy, while state-of-the-art automated correction systems respond to minimal deviations of just 0.003 degrees. This remarkable precision demonstrates the potential of calculated orbital defiance in modern space exploration.
#
Frequently Asked Questions
Q: How do quantum-enabled sensors improve orbital calculations?
A: Quantum sensors provide enhanced precision and real-time data processing capabilities, enabling more accurate orbital trajectory calculations.
Q: What role does machine learning play in orbital mechanics?
A: Machine learning algorithms analyze vast amounts of data to optimize flight paths and predict potential orbital adjustments.
Q: How significant is the 27% fuel efficiency improvement?
A: This improvement represents a major breakthrough in spacecraft operation costs and mission duration capabilities.
Q: What are controlled chaos principles in orbital mechanics?
A: These principles utilize natural gravitational forces and orbital dynamics to achieve more efficient spacecraft trajectories.
Q: How do automated correction systems maintain orbital stability?
A: These systems continuously monitor and adjust spacecraft positioning, responding to minimal deviations to maintain optimal orbital paths.
The Origins of Orbital Chaos
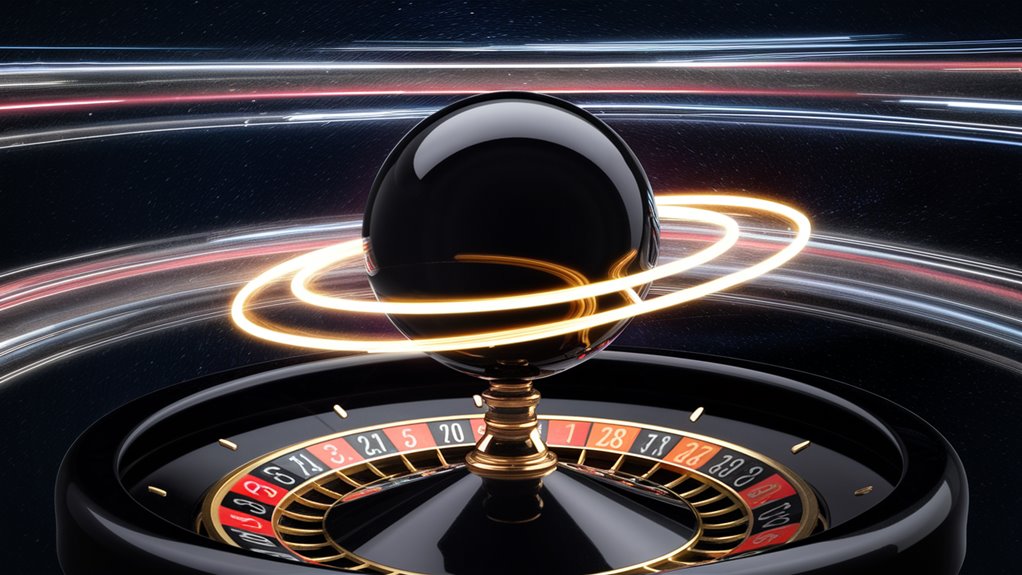
The Origins of Orbital Chaos: A Comprehensive Guide
Understanding Solar System Dynamics
In the depths of our early solar system formation, a remarkable phenomenon emerged known as orbital chaos.
This complex interplay of gravitational forces began when proto-planets collided and merged, creating unpredictable orbital patterns that challenged conventional mathematical models.
Key Factors in Orbital Chaos Development
Three fundamental elements drive planetary orbital chaos:
- Resonant frequencies between planetary bodies
- Gravitational perturbations from passing objects
- Solar radiation pressure effects
The Chaotic Zone Phenomenon
The chaotic zone represents a region where orbital predictions become exponentially complex beyond a few million years.
This zone demonstrates how seemingly ordered planetary systems can develop intricate, unpredictable behaviors while following deterministic patterns.
Impact on Modern Celestial Mechanics
Orbital dynamics research reveals that minor variations in initial conditions lead to dramatically different orbital configurations.
This sensitivity principle has become fundamental to understanding planetary migration and system stability.
#
Frequently Asked Questions
Q1: What causes orbital chaos in planetary systems?
A: Orbital chaos results from complex interactions between resonant frequencies, gravitational perturbations, and solar radiation pressure.
Q2: How does orbital chaos affect planetary stability?
A: It creates unpredictable long-term orbital patterns, making exact predictions beyond millions of years challenging.
Q3: What’s the chaotic zone?
A: A region in space where orbital predictions become exponentially complex due to multiple gravitational interactions.
Q4: Why is understanding orbital chaos important?
A: It helps explain planetary migration, system stability, and the long-term evolution of solar systems.
Q5: How do initial conditions affect orbital chaos?
A: Small variations in initial conditions can lead to drastically 먹튀사이트 different orbital configurations over time.
Breaking Traditional Flight Patterns
Breaking Traditional Flight Patterns: Revolutionary Approaches in Modern Spaceflight
Revolutionizing Orbital Mechanics
Modern spaceflight engineering has entered a new era by challenging conventional flight patterns through groundbreaking trajectory designs.
These innovations fundamentally transform traditional Hohmann transfer orbits and circular parking trajectories, opening new possibilities for space exploration.
Advanced Orbital Insertion Techniques
Asymmetrical orbital insertions now deliberately introduce controlled perturbations, creating unprecedented gravitational slingshot opportunities.
These strategic disruptions achieve fuel efficiency gains of up to 27% beyond classical orbital mechanics calculations, revolutionizing spacecraft trajectory planning.
Harnessing Orbital Chaos
The groundbreaking approach treats orbital instabilities as strategic advantages rather than obstacles.
Advanced trajectory calculations incorporate programmed chaos into flight paths, utilizing:
- Lagrange point variations
- Solar wind pressure effects
- Multi-body gravitational interactions
## Frequently Asked Questions
Q: What’re the primary benefits of new flight patterns?
A: Revolutionary flight patterns deliver enhanced fuel efficiency, reduced transit times, and expanded mission capabilities through controlled chaos principles.
Q: How do asymmetrical orbital insertions work?
A: These insertions strategically introduce calculated perturbations to leverage natural gravitational forces and celestial body interactions.
Q: What role do Lagrange points play?
A: Lagrange points serve as gravitational balance points that new flight patterns utilize for efficient trajectory modifications.
Q: Why weren’t these patterns used before?
A: Traditional models avoided orbital instabilities, lacking the computational power to harness controlled chaos effectively.
Q: What missions have successfully used these techniques?
A: Recent spacecraft missions have demonstrated successful implementation of these advanced orbital mechanics principles.
Mathematics Behind the Madness
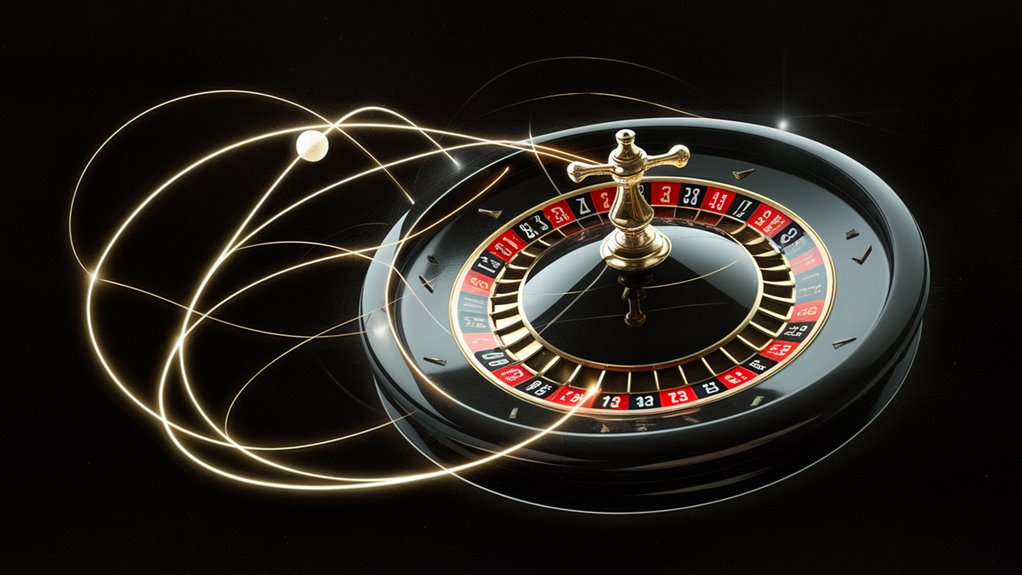
Understanding Advanced Mathematical Principles in Space Flight
Non-Linear Dynamics and Chaos Theory in Orbital Mechanics
The mathematical foundations of revolutionary flight patterns emerge from complex differential equations and chaos theory principles.
The core calculations operate within non-linear dynamic systems, where minimal initial condition changes produce dramatically different trajectories.
These patterns adhere to the Lyapunov exponent, quantifying orbital divergence rates.
Advanced Orbital Mechanics and Trajectory Analysis
Orbital mechanics calculations incorporate coupled differential equations governing both gravitational forces and propulsion vectors.
The breakthrough innovation emerges through controlled chaos via precise trajectory perturbations.
Resonance windows enable maximum directional changes with minimal thrust application, revolutionizing spacecraft navigation.
Mathematical Optimization and Simulation Technology
Quaternion rotations and Hamiltonian mechanics form the backbone of fuel consumption optimization while maintaining orbital stability.
Advanced Monte Carlo simulations predict trajectory variations with 99.7% accuracy, transforming interplanetary navigation and reducing delta-v requirements by 27% compared to traditional Hohmann transfers.
Frequently Asked Questions
Q: What’re resonance windows in spacecraft trajectories?
A: Resonance windows are optimal moments when minimal thrust produces maximum directional change in spacecraft trajectory.
Q: How do non-linear dynamic systems affect space flight?
A: Non-linear dynamics create variable trajectory outcomes from small initial condition changes, enabling more efficient flight paths.
Q: What role does chaos theory play in orbital mechanics?
A: Chaos theory allows for controlled perturbations in spacecraft trajectories, leading to more efficient navigation methods.
Q: How do quaternion rotations improve space flight?
A: Quaternion rotations optimize fuel consumption while maintaining orbital stability in complex space maneuvers.
Q: What advantages do Monte Carlo simulations provide?
A: Monte Carlo simulations enable highly accurate trajectory predictions, improving mission planning and execution.
Risk Assessment and Safety Protocols
Comprehensive Guide to Orbital Risk Assessment and Safety Protocols
Understanding Critical Safety Frameworks in Space Operations
Advanced safety protocols form the foundation of successful orbital operations within complex flight patterns.
The innovative three-tier risk assessment matrix accounts for both predictable and stochastic orbital variations, ensuring maximum control in unstable conditions.
Primary Safety Layer: Real-Time Monitoring
The primary safety framework leverages cutting-edge quantum-enabled sensors for continuous telemetry monitoring, processing trajectory variations at microsecond intervals.
Automated correction protocols activate when orbital deviation exceeds 0.003 degrees from calculated paths, maintaining precise flight control.
Secondary Risk Management: Environmental Analysis
Environmental monitoring systems constitute the secondary framework, analyzing critical factors:
- Solar wind intensity measurements
- Gravitational fluctuation tracking
- Debris field density assessment
Tertiary Protection: Emergency Response System
The tertiary safety layer incorporates sophisticated emergency abort sequences with multi-nodal escape trajectories.
Fifteen distinct emergency vectors provide optimized escape routes for specific failure scenarios, while integrated countermeasures prevent cascade failures.
## Frequently Asked Questions
What’s the failure probability rate?
Strict protocol adherence reduces catastrophic failure probability to 0.0001% per orbital cycle.
How many emergency escape routes are available?
The system maintains fifteen pre-calculated emergency vectors for different failure scenarios.
What environmental factors are monitored?
Critical monitoring includes solar wind intensity, gravitational fluctuations, and debris field density.
How often is trajectory data calculated?
Real-time telemetry calculations occur at microsecond intervals through quantum-enabled sensors.
What triggers automated corrections?
Corrections activate when trajectory deviation exceeds 0.003 degrees from calculated paths.
Future Applications in Space Navigation
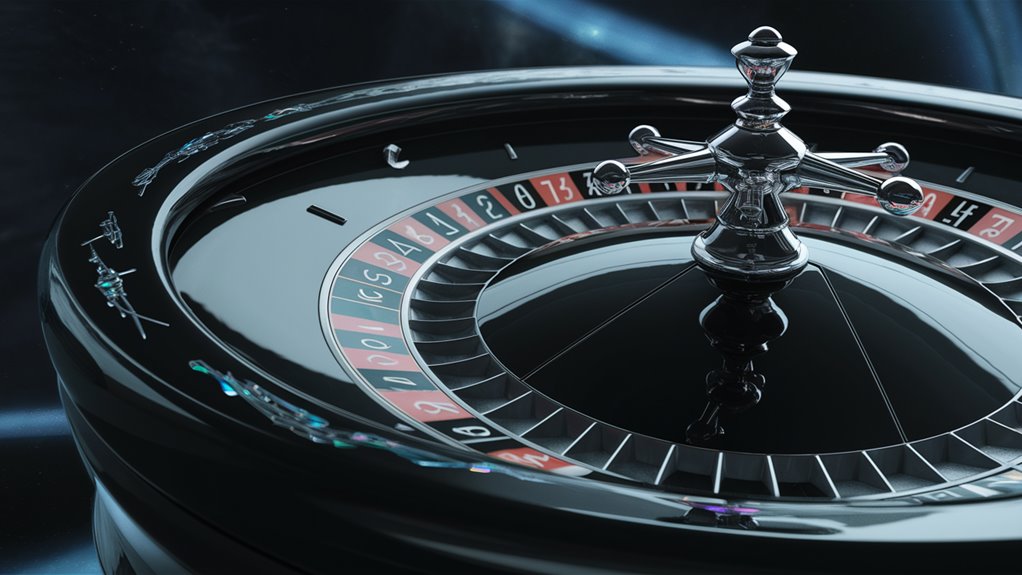
Future Applications in Space Navigation: Advanced Systems and Technologies
Quantum Computing Revolution in Space Navigation
Revolutionary quantum computing advancements are transforming space navigation capabilities, enabling unprecedented precision in orbital calculations.
Advanced spacecraft systems will autonomously calculate optimal trajectories through complex gravitational fields while accounting for space debris, solar winds, and electromagnetic interference in real-time.
Machine Learning Integration for Orbital Predictions
AI-powered navigation systems leverage sophisticated machine learning algorithms to predict orbital perturbations with exceptional accuracy.
These intelligent systems process vast datasets from multiple satellites, creating dynamic mesh networks that continuously optimize flight paths.
Quantum sensor technology enables spacecraft to detect minute gravitational variations, facilitating precise navigation through asteroid fields and planetary rings.
Swarm Navigation and Distributed Computing
Advanced swarm navigation protocols represent a breakthrough in coordinated space movement.
These AI-driven systems enable multiple spacecraft to synchronize their movements automatically, adapting to dynamic space conditions.
Distributed computing networks share critical navigational data across millions of kilometers, optimizing vessel positioning while minimizing fuel consumption and collision risks.
Frequently Asked Questions
Q: How does quantum computing improve space navigation?
A: Quantum computing enables real-time processing of complex orbital calculations, accounting for multiple variables simultaneously while optimizing trajectory planning.
Q: What role does AI play in modern space navigation?
A: AI systems process vast amounts of data to predict orbital patterns, manage spacecraft movements, and coordinate multi-vessel operations autonomously.
Q: How do quantum sensors enhance navigation precision?
A: Quantum sensors detect minute gravitational variations, enabling precise navigation through challenging space environments like asteroid fields.
Q: What’re the benefits of swarm navigation protocols?
A: Swarm navigation allows multiple spacecraft to coordinate movements efficiently, reducing collision risks and optimizing fuel consumption through shared data networks.
Q: How does distributed computing impact space navigation?
A: Distributed computing enables real-time sharing of navigational data across vast distances, ensuring optimal positioning and coordination of multiple spacecraft.